#IARC2017 – The Science Behind Several New Strategies for Treating Friedreich’s Ataxia
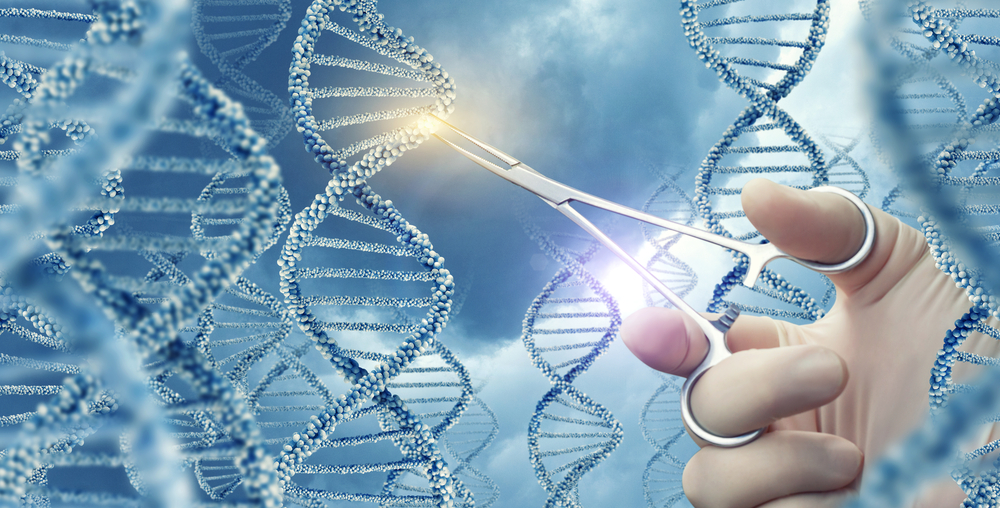
Dozens of scientists from universities and pharmaceutical companies will come together at the 2nd International Ataxia Research Conference in Pisa, Italy, Sept. 27-30 to discuss new strategies for addressing the underlying mechanisms of ataxias.
The conference will focus in particular on inherited ataxias, or those with a genetic cause. Since Friedreich’s ataxia (FA) is the most common inherited form, it will draw the lion’s share of attention.
FA is caused by mutations of the FXN gene, which codes for the production of the frataxin protein. This protein is found in several cells, but has higher levels of expression in the heart, spinal cord, liver, pancreas, and the skeletal muscles that control movement.
The nervous systems of people with FA are damaged, leading to impaired muscle coordination — or ataxia — that worsens over time.
A person’s genome is their complete set of DNA, including all of their genes. The genome is composed of four building blocks of life known as nucleotides: adenine (A), cytosine (C), guanine (G), and thymine (T). A normal FXN gene has a region composed of three nucleotides – GAA – that are repeated between five and 33 times.
In FA patients, the number of GAA trinucleotide repeats is abnormally high — from 66 to more than 1,000 times — in both copies of the gene. FA is called a genetic recessive disease because the condition manifests itself only in people who inherit two defective copies of the FXN gene, one from their father and one from their mother.
Since FA is caused by mutations of a single gene, scientists are pursuing gene therapy, RNA therapy and gene editing strategies, such as CRISPR, as potential treatments for the condition.
Gene therapy involves introducing new genetic material into specific cells to correct an existing genetic abnormality. Scientists do this either by replacing a mutated gene with a healthy copy — an approach known as gene augmentation therapy — or by inactivating the gene that is not working properly, an approach known as gene inhibition therapy.
Another gene therapy strategy is not to correct the underlying genetic defect, but to introduce a new gene that helps fight the disease.
Regardless of the type of gene therapy used, the strategy relies on transferring a DNA sequence — which is a component of a gene — or a gene itself.
Scientists can use different vehicles, or vectors, to deliver genetic information to cells. They pack the information in viral or non-viral delivery systems. Some of the non-viral delivery systems are physical ones and some are chemical ones.
A viral system involves delivering a new gene while infecting a cell. Scientists must modify the delivery viruses to prevent them from causing a disease. Some of the delivery viruses they use include retrovirus, adenovirus, adeno-associated virus, herpes simplex virus, pox virus, lentivirus, and Epstein–Barr virus.
Each vector system has advantages and limitations. For example, retroviral vectors can permanently integrate into the genome of the cell they infect. But for the DNA they are carrying to take hold, the cells must be dividing. In contrast, adenoviral vectors can deliver genes to both dividing and non-dividing types of cells; but these cells are more vulnerable to being eliminated by the immune system.
Physical methods for delivering nonviral systems include gene guns, electroporation, particle bombardment, ultrasound, and magnetofection. Chemical delivery methods include cationic liposomes and polymers.
Non-viral systems are less effective at delivering DNA than viral systems. But they can be made in larger quantities, are more cost-effective, and rev up the immune system less than viral systems.
Key challenges that researchers face in gene therapy include delivering a gene to the right place and activating it, preventing the body from generating an immune response to the gene, and making sure that the newly inserted DNA does not disrupt the function of the cell’s other genes. Another challenge is the high cost of the therapy.
Scientists are developing several gene therapy approaches to treating FA. They target either the nervous system or cardiac features of the disease.
Researchers are also looking at RNA-based therapies for FA. Our cells carry three types of RNA molecules: messenger, ribosomal, and transfer RNA.
Messenger RNA, or mRNA, are molecules that carry information encoded in DNA. The process by which a DNA molecule is copied into an RNA molecule is called transcription.
After transcription, cell components called ribosomes convert the information of the mRNA into proteins. Converting mRNA information to proteins is called translation.
In most cases of FA, the GAA-repeat expansion leads to transcriptional silencing — that is, it halts or severely diminishes the production of mRNA. This, in turn, results in insufficient production of frataxin protein.
One RNA therapy for delivering functional frataxin mRNA to ribosomes is called RNA transcript therapy, or RTT. It involves using lipid nanoparticles to deliver frataxin mRNA to cells.
Another RNA-based strategy is to target the mechanism by which excessive GAA repeats silence transcription. The excessive GAA repeats in FA patients’ mRNA frataxin molecules lead to the formation of an R-loop between the DNA molecule and the new mRNA molecule. The loop interferes with the production of mRNA, reducing the amount of frataxin that is generated.
In order to target RNA-DNA binding and block R-loop formation, scientists are developing synthetic RNA-based molecules – duplex RNAs and locked nucleic acid (LNA) oligonucleotides – that can block GAA repeats in newly formed frataxin mRNA. The idea behind this approach is re-activating transcription.
In recent years, researchers have also developed gene-editing strategies that allow them to repair, replace, or modify genes. These approaches offer great potential for treating diseases caused by gene mutations, including FA.
The latest members of the growing family of genome editing systems are the Transcription Activator-Like Effector Nucleases, or TALENs, and the Clustered Regularly Interspaced Short Palindromic Repeats/Cas9, or CRISPR/Cas9.
Both use specialized proteins to remove excessive GAA repeats from DNA, but do it in different ways.
You can think of both the TALEN and CRISPR/Cas9 gene-editing strategies as molecular scissors that cut GAA repeats in the FTX gene, restoring the proper expression of the gene and thus the production of frataxin protein.
The TALENS approach involves using TAL effector proteins secreted by the plant bacteria Xanthomonas to bind to DNA so that cuts can be made.
In contrast, the CRISPR/Cas9 approach is based on a strategy that the immune systems of many bacteria use to protect themselves from outside genetic elements. It involves using short RNA sequences – or RNA guides – composed of 20 nucleotides to target a DNA sequence. Once they bind to the DNA, the nuclease Cas9 can cut the sequence.
Friedreich’s Ataxia News will be covering the IARC meeting, offering readers daily glimpses at the latest advances and discoveries in ataxia research. For more information about the meeting, please read our related story and interviews with key leaders in the ataxia field.